Tesera Trabecular Technology® is an advanced highly porous structure designed and built to support bone in-growth while providing long-term stability and strength.
Read more in the Tesera Trabecular Technology Technical Brochure (PDF).
Applications
Advanced materials for enhanced device performance
KYOCERA is committed to providing innovative products that help surgeons meet demanding patient needs. By combining advanced materials, like the Tesera Trabecular Technology porous structure, with tried-and-true design principles, KYOCERA aims to enhance device performance and longevity. Tesera Trabecular Technology implants provide:
- The initial stability required for early fixation
- The strength required for weight-bearing,
- A scaffold for bone in-growth and long-term fixation
![]() |
![]() |
Tesera Trabecular Technology Acetabular System | Tesera Trabecular Technology Stand-Alone ALIF System |
Tesera Trabecular Technology porous structure is highly porous, with an average pore diameter of about 500µm.[15] Pores of this size can accommodate bone in-growth and the vascularization required to sustain living bone.[8-11]
Specifications
Designed for bone in-growth
Each parameter of the Tesera Trabecular Technology porous structure—from pore size and shape to surface roughness—was design based on decades of published research on bone in-growth surfaces.
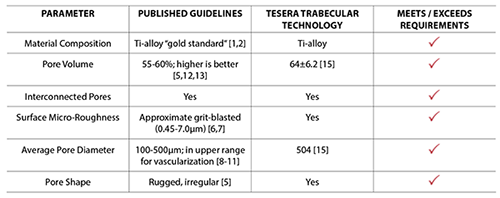
![]() |
Animal Study
Proven biocompatibility
Roughened titanium alloy has been used clinically for more than 35 years and remains the gold standard for bone on-growth.[1,2] A study of bone in-growth into Tesera Trabecular Technology bone plugs was conducted in a sheep femur model.[16,26] The results revealed:
- No implant-associated adverse effects on the host bone
- Excellent new bone formation
- Bone remodeling within and adjacent to the porous structure
![]() |
Light microscope images showing excellent bone ingrowth by the 12 week time point and continued bone growth at 24 weeks.[16] |
![]() |
Mechanical Properties
Strength and bone-like modulus for long-term success
![]() |
The Tesera porous structure is not a coating; the solid and porous portions are built up in one continuous process. This process produces 100% dense titanium alloy, with properties like wrought material.[23] |
![]() |
The modulus of the Tesera porous structure falls within the range of values reported for cancellous bone. |
Stability
Initial stability for secure fixation
![]() |
The large pore size of the Tesera porous structure provides initial mechanical stability as the surface prominences grip into the bone; the mechanical interlock of bone growing into the structure provides long-term fixation. |
![]() |
In laboratory testing of the Tesera structure on cancellous bone, the coefficient of friction was substantially improved over plasma-strayed coating and better than a contemporary highly porous tantalum structure.[18-20] A higher frictional coefficient enhances initial stability and promotes ingrowth by limiting micromotion at the bone-to-implant interface.[21,22] |
- Oshida Y. Bioscience and bioengineering of titanium materials. Oxford: Elsevier. 2012.
- Kieswetter K, et al. The role of implant surface characteristics in the healing of bone. Crit Rev Oral Biol Med. 1996; 7(4):329-345.
- Arcelli D, Palmieri A, Pezzetti F, Brunelli G, Zollino I, Carinci F. Genetic effects of titanium surface on osteoblasts: A meta-analysis. J Oral Sci. 2007 Dec;49(4):299-309.
- Mour M, et al. Advances in Porous Biomaterials for Dental and Orthopaedic Applications. Materials. 2010;3:2947-2974.
- Nouri A, Hodgson PD, Wen C. Biomimetic Porous Titanium Scaffolds for Orthopedic and Dental Applications. In Mukerjee A, ed. Biomimetics Learning from Nature. InTech 2010;21:415–450.
- Hacking SA, Bobyn JD, Tanzer M, Krygier JJ. The osseous response to corundum blasted implant surfaces in a canine hip model. Clin Orthop Relat Res. 1999 Jul;(364):240-53.
- Feighan JE, Goldberg VM, Davy D, Stevenson S. The influence of surface-blasting on the incorporation of titanium-alloy implants in a rabbit intramedullary model. JBJS Am. 1995; 77:1380-1395.
- Simske SJ, Ayers RA, Bateman TA. Porous materials for bone engineering. Mater Sci Forum. 1997;250:151–182.
- Bobyn JD, Pilliar RM, Cameron HU, Weatherly GC. The optimum pore size for the fixation of porous-surfaced metal implants by the ingrowth of bone. Clin Orthop Relat Res. 1980 Jul-Aug;(150):263-70.
- Miao X, Sun D. Graded/Gradient Porous Biomaterials. Materials. 2010;3:1-22.
- Bansiddhi A, Sargeant TD, Stupp SI, Dunand DC. Porous NiTi for bone implants: A review. Acta Biomater. 2008 Jul;4(4):773–782.
- Bragdon CR, Jasty M, Greene M, Rubash HE, Harris WH. Biologic Fixation of Total Hip Implants. Insights gained from a series of canine studies. JBJS Am. 2004;86-A Suppl 2:105-117.
- Karageorgiou V, Kaplan D. Porosity of 3D biomaterial scaffolds and osteogenesis. Biomaterials. 2005 Sep;26(27):5474-91.
- Data on file with KYOCERA Medical Technologies, Inc. SEM Evaluation. Test Report K13047307-1.
- Data on file with KYOCERA Medical Technologies, Inc. Coating Evaluation per ASTM F1854-09. Test Report K12076592-4.
- Surgeries were performed at IMDS Discovery Research (Logan, Utah); processing and analysis of the specimens was conducted by the Bone and Joint Research Laboratory (Salt Lake City, Utah). Data on file with KYOCERA Medical Technologies, Inc.
- Willie BM, Bloebaum RD, Bireley WR, Bachus KN, Hofmann AA. Determining relevance of a weight-bearing ovine model for bone ingrowth assessment. J Biomed Mater Res A. 2004 Jun;69(3):567-76.
- Data on file with KYOCERA Medical Technologies, Inc. Coefficient of Friction ASTM G115-10. Test Report K13047307-4.
- Shirazi-Adl A, Dammak M, Paiement G. Experimental determination of friction characteristics at the trabecular bone/porous–coated metal interface in cementless implants. J Biomed Mater Res. 1993;27(2):167–75.
- Zhang Y, Ahn PB, Fitzpatrick DC, Heiner AD, Poggie RA, Brown TD. Interfacial frictional behavior: cancellous bone, cortical bone, and a novel porous tantalum biomaterial. Journal of Musculoskeletal Research. 1999;3(4):245-251.
- Dammak M, Shirazi-Adl A, Schwartz M Jr, Gustavson L. Friction properties at the bone–metal interface: comparison of four different porous metal surfaces. J Biomed Mater Res. 1997 Jun; 35(3):329–336.
- Biemond JE, Aquarius R, Verdonschot N, Buma P. Frictional and bone ingrowth properties of engineered surface topographies produced by electron beam technology. Arch Orthop Trauma Surg. 2011 May;131(5):711-8.
- Hiemenz J. EBM offers a new alternative for producing titanium parts and prototypes. Time-Compression Technologies. 2006 May/June: 16-20.
- asty M, Bragdon C, Burke D, O’Connor D, Lowenstein J, Harris W. In vivo skeletal responses to porous-surfaced implants subjected to small induced motions. JBJS Am. 1997 May;79(5):707-14.
- Data on file with KYOCERA Medical Technologies, Inc. Tensile/Compression Tests. Test report K13047307-5.
- Willie BM, Bloebaum RD, Bireley WR, Bachus KN, Hofmann AA. Determining relevance of a weight-bearing ovine model for bone ingrowth assessment. J Biomed Mater Res A. 2004 Jun 1;69(3):567-76.
*Trabecular Metal is a trademark of Zimmer, Inc. (Warsaw, Indiana).
Distributor Inquiry